In traditional collider physics experiments, the realms of accelerator and particle physics are largely separated. For most particle physicists, the details of the particle beam dynamics can just be reduced to a few numbers indicating the beam energy, beam luminosity and beam size at the collider interaction point(s). For smaller-scale precision measurements this separation can be much less clear.
The Fermilab Muon g-2 experiment, which has recently released their latest measurements of the anomalous magnetic moment of the muon, is a topical example where beam dynamics is intrinsically entwined with the physics measurement being made, and the distinction between accelerator and particle physicists is blurred.
The Fermilab Muon g-2 experiment measurement campaign ran from 2018 to Summer 2023, with three years of this data yet to be fully analysed. At its heart the g-2 experiment had an anti-muon beam circulating in the highly-uniform 1.45T dipole field of the storage ring, with vertical containment provided by electrostatic quadrupoles. The energy spectrum and tracks of positrons coming from the decays of the anti-muons in the beam were reconstructed from calorimeters and straw trackers located on the inside of the ring. The chiral nature of the weak force meant that the positron trajectories and measured energies varied periodically according to the direction of the precessing spin vectors of the anti-muons. Hence, the rate of spin precession could be reconstructed, and ultimately the gyromagnetic ratio of the anti-muon determined.
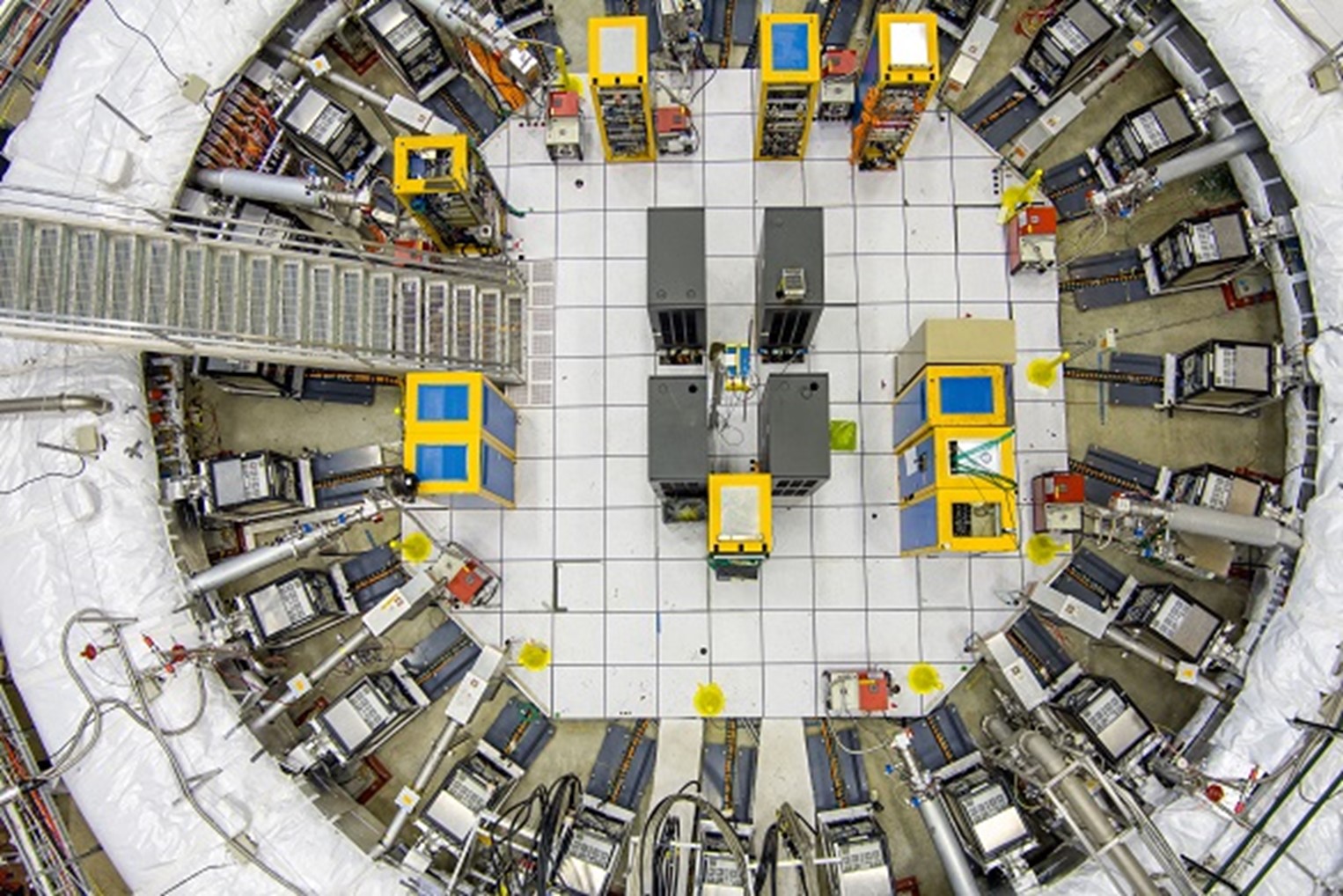
Dr Ian Bailey, lecturer at Lancaster University and a member of the Cockcroft Institute in the UK, is one of the accelerator scientists who contributed to the experiment. He said: “It is important to understand both the starting anti-muon phase space distribution, and the details of how it evolves under the influence of the inflector magnet, kicker magnet, quadrupole fields - including fringe fields - and minor imperfections in the dipole field.”
Many groups from different institutes work on analysing these effects as part of the Fermilab Muon g-2 collaboration. Dr Ian Bailey and his colleagues at the Cockcroft Institute have mostly contributed to the simulations of the anti-muon beamlines. These simulations start where the 8 GeV proton bunches from the Fermilab recycler synchrotron are directed to a nickel-iron target producing a mix of predominantly protons, pions and muons which is collected using a lithium lens. Positively-charged particles with momenta close to the "magic momentum" of 3.1 GeV/c needed for the experiment are selected by using a bending magnet. As the positive pions travel through the beamlines they decay, producing anti-muons with ∼95 % polarization.
The beam is then injected into the delivery ring where almost all remaining pions decay into muons while the heavier protons lag behind the pions and muons and are mostly removed by timing of the kicker magnet. The beam continues through over 100 m of beamline before entering the muon storage ring at an optimised angle though a narrow channel in the dipole field of the storage ring created by a superconducting inflector magnet.
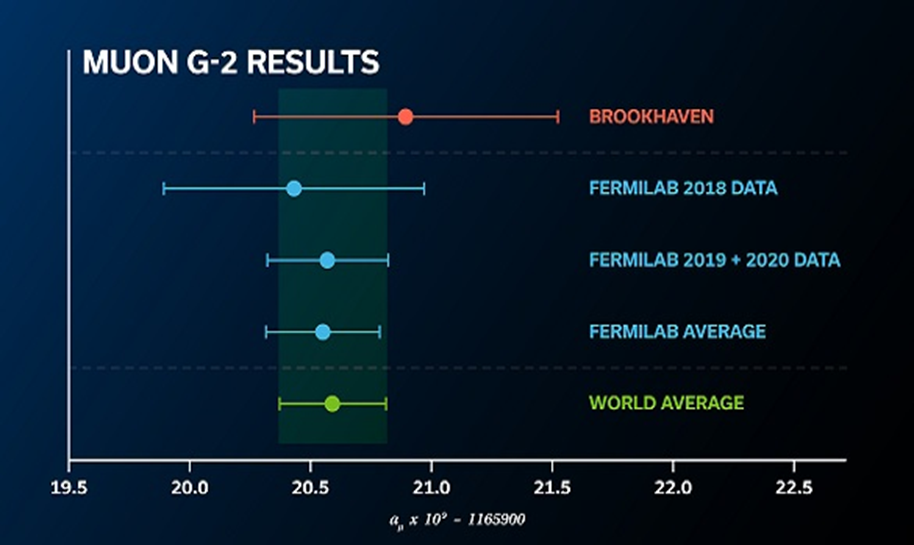
Simulations through the beamlines as well as those inside the storage ring are essential, as the measurement itself is exquisitely sensitive to the details of the anti-muon distribution in phase space; the target sensitivity of 140 ppb requires a detailed understanding of the correlations between the phase space coordinates. For example, the decay rate and spin distributions of the anti-muons depend on their starting energy distributions, which combine to give a time-dependence to the spin distribution being measured by the experiment. This effect is one of the smaller ones that need to be accounted for. More dominant effects come from considering the effects of the anti-muons moving through the electrostatic quadrupole field. The quadrupoles introduce vertical betatron oscillations which give an extra contribution to the spin precession in the dipole field. In addition, as the anti-muons move through the radial electric field formed by the electrostatic quadrupoles they experience an additional momentum-dependent, vertically-oriented magnetic field. Other corrections come from considering whether the spin distribution of anti-muons being lost to collimation are representative of the spin distribution of the whole anti-muon population and from looking at how the acceptance of the detectors depends on the spin phase of the anti-muons which correlates with the time-dependent spatial distribution of the beam.
The simulations that feed into many of these studies will continue to be refined as the analysis of the remaining data continues. The interplay of the beam dynamics and the physics measurement which is sensitive to the existence of new physics beyond the Standard Model provides a rich environment for the exchange of ideas between groups who often work separately in other contexts.
David Tarazona, postdoctoral associate at Cornell University (USA) and one of the coordinators of the beam dynamics activities, added: “Our collaboration has conducted a unique effort to orchestrate seemingly incompatible branches of physics for achieving a measurement to outstanding precision. Beam physics is one essential ingredient, where a deep understanding of the muon beam dynamics allows us to achieve such a wonderful measurement. We are excited to continue working together for the next two years to share our final results with the physics community.”
Link to Youtube:
Muon g-2 experiment returns with new precision measurement: https://youtu.be/hkHd_wxMfrs
Further reading:
Muon g-2 Collab. 2023 https://muon-g-2.fnal.gov/result2023.pdf
Muon g-2 Collab. 2021 Phys. Rev. Lett. 126 141801
BMW Collab. 2021 Nature 593 51
Muon g-2 Theory Initiative 2020 Phys. Rept. 887 1
Fermilab is America’s premier national laboratory for particle physics research. For further information, visit Fermilab’s website (https://www.fnal.gov/).