Already now the large majority of the almost 40,000 particle accelerators in operation worldwide are used in industry and medicine, and this number is rapidly increasing [1]. Accelerator applications are progressing fast, and particle accelerators have the potential to become a crucial tool in the ongoing 4th industrial revolution, making accessible to industry and medicine processes that allow a direct interaction with the atomic and subatomic structure of materials and cells. But to succeed, this “industrial revolution” needs small accelerators that can easily fit in a medical or industrial environment, easy to operate with moderate energy requirements and minimum radiation concerns. In short, what is needed are “miniature accelerators”, for the moment still a technological dream although several accelerator teams are heading in this direction. Every technology starts from a dream, but how far are we from realising it?
The first important consideration is that size is not all. The basic parameters of an accelerator can be divided in two categories, those defining the “performance” for the final users (type of particle, energy, beam current, beam brightness, reliability) and those defining the “impact” on the operating environment (mains power, power efficiency, radiation emission and activation, dimensions, weight, construction and operation costs). The real challenge for the miniature accelerator consists in maximizing the first set of parameters, minimising the second one. Every “miniature” device must provide a minimum performance that will make the system attractive to its potential users.
Lighter, smaller, cheaper: Compacting the convention
The first direction to reduce the size of our accelerators is “incremental” innovation, pushing to its limits the good old concept of radiofrequency (RF) acceleration that since almost 100 years drives the particles within our accelerators.
In the field of proton acceleration, this translates into miniaturising the traditional “workhorses” of low-energy acceleration: the Radio Frequency Quadrupole (RFQ) and the cyclotron. Several developments are going on towards high-frequency compact RFQ’s [2]; while the RFQ gradient can reach some 2-3 MeV/m, the main limitation comes from the small aperture and limited cooling capability that set a limit to the average beam current. Very compact cyclotrons in most cases superconducting are also a popular trend [3]. Here the average current can be higher, and the final energy is of the order of 5 MeV/m2 – taking the surface of the accelerator as a reference instead of its length! In terms of cost, for both cases the driver is the ancillary equipment: the RF generator for the RFQ, and the cryogenic system for the superconducting cyclotron.
For electrons instead, the driving incremental development has been the decade long R&D work done by the CLIC team to push the gradient of X-band structures. Reaching some 100 MeV/m (corresponding to some 20 MeV/m2…), X-band technology is now proposed for compact Free Electron Lasers, X-ray source, FLASH cancer treatment, etc [4].
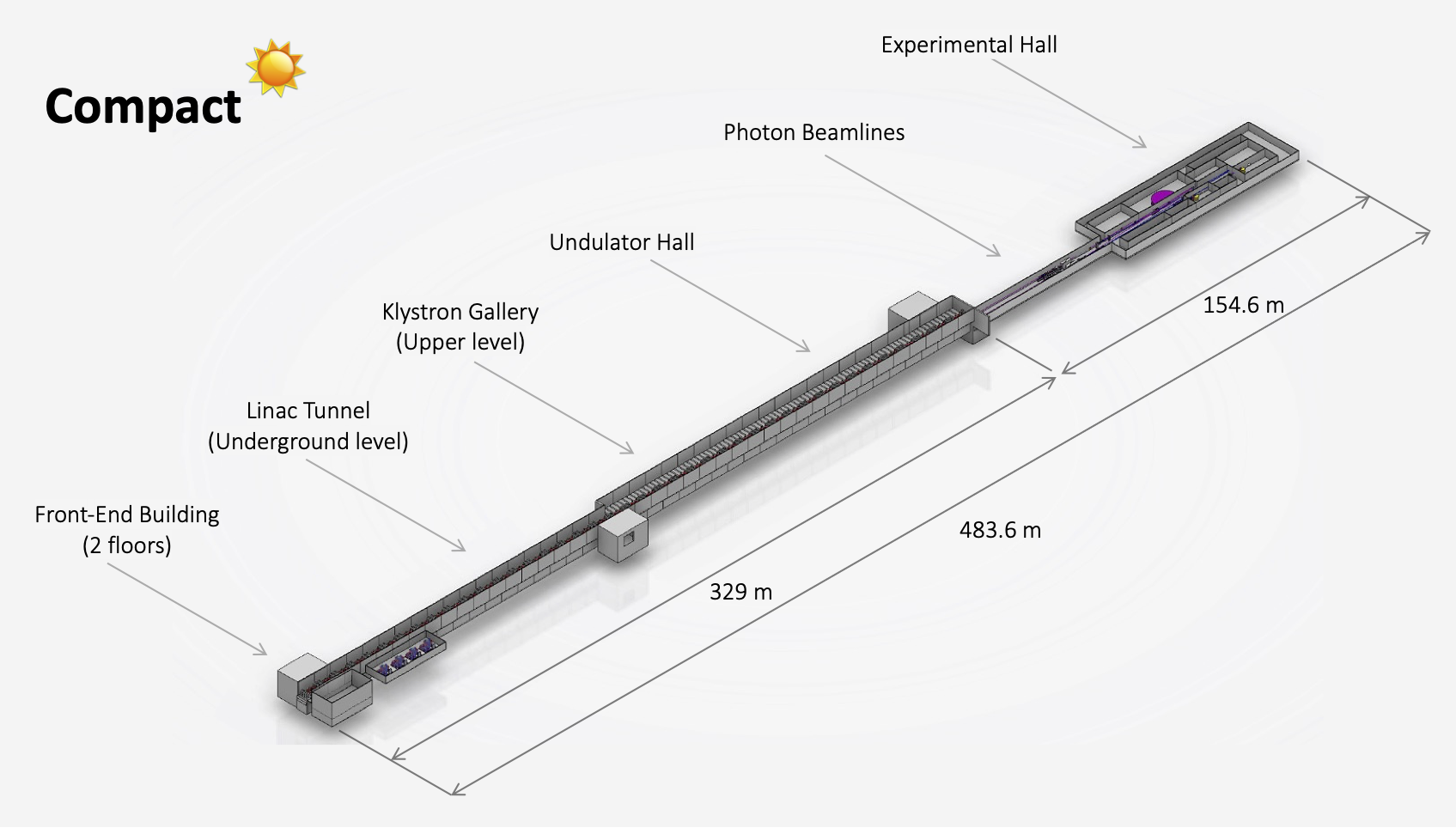
Accelerators in a shoe-box?
An alternative very popular avenue to miniaturise accelerators comes from “disruptive” innovation that might eventually completely replace RF technology with lasers that provide energy to the particles using either plasmas or dielectric structures for the energy conversion. Here the technological landscape is very wide, but the challenges to face are still huge. Much experimental work is going in the direction of proton and ion laser-based acceleration to some MeV of energy, but critical issues that remain to be solved are beam quality and reproducibility. The energy is there, but the beam is still very far from what users need. Progress with electron acceleration is more promising, with the advantage for compact accelerators of being easily single-stage, free from the difficult problem of multi-stage acceleration that has still to be solved for high-energy acceleration.
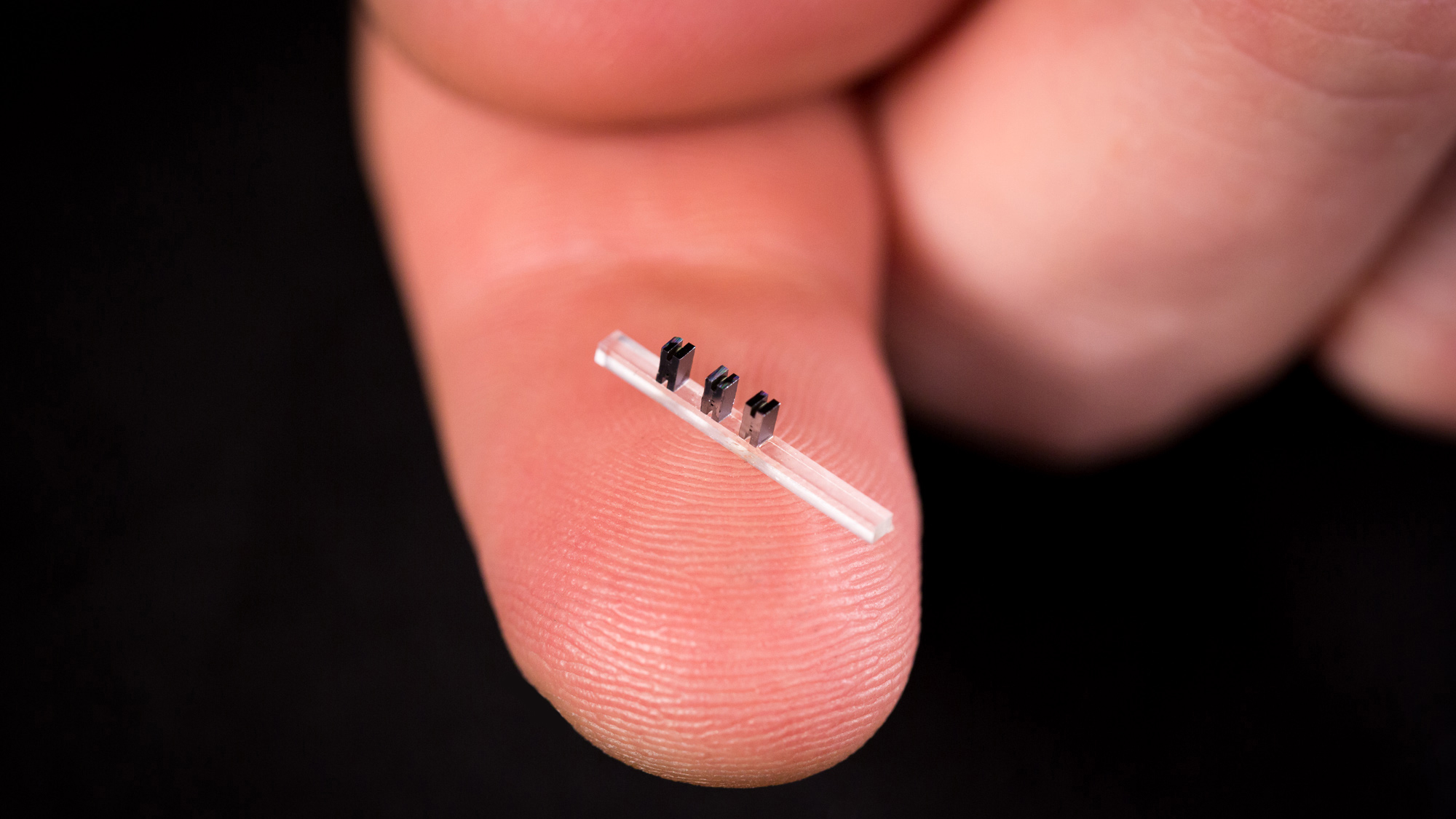
The way to communicate these projects is also very attractive, as for the “accelerator in a shoe-box”, aiming at accelerating electrons through a ridged silicon glass chip fed by a laser [4]. The project is producing its first results, but again the challenge is to push enough particles to be of some use through such a miniaturised structured. These developments often aim at medical application as first goal (as an accelerator in a catheter!), but if it is true that medical applications don’t need high currents, it is also true that this is the domain with the most stringent requirements in terms of beam quality and stability.
In conclusion, accelerator science is progressing, but we are still far from having an accelerator that can fit in every small workshop and every medical ward. For the moment, dreams remain dreams, but there is some rapidly progressing reality in them. While the real “miniature” is still faraway, compact accelerators tailored to specific usages are at reach, and may benefit from some targeted R&D (additive manufacturing, high RF frequencies, solid-state RF technology) in the incremental direction, and from the fast development of powerful lasers and miniaturised chips in the disruptive direction. New accelerator applications are appearing, in particular in medicine, industry, and environment, opening new potential users and commercial markets for the new technologies that will come out of the quest for the miniature accelerator!
[1] See for example the comprehensive study “Applications of Particle Accelerators in Europe” published by the EuCARD2 project, available at http://apae.ific.uv.es/apae/wp-content/uploads/2015/04/EuCARD_Applications-of-Accelerators-2017.pdf
[2] See for example for small-scale accelerators for cancer treatment and to study heritage artworks.
[3] AMIT project of CIEMAT.
[4] See for example this project to mount “accelerators on a chip” to boost the energy of electrons.