In the wake of the CLIC conceptual design study in 2011, an exciting R&D initiative emerged, dedicated to non-invasive beam diagnostic tools tailored for highly relativistic short bunches. This journey has traversed continents, spanning from the United States to Japan and the halls of CERN and UK laboratories (Diamond Light source and Daresbury).
It all started from a collaboration between the Royal Holloway University of London and CERN, spurred by the quest for alternatives to the costly and intricate laser wire scanner, looking for solutions capable of mesuring micron scale beam sizes [1]. Thus, a young colleague embarked in 2011 on a Ph.D. study with the aim to develop a non-invasive monitor based on the emission of incoherent Diffraction Radiation (DR) emitted by charged particles as they travel through a thin slit made out of dielectric material [2].
In 2012, a detector materialised, finding its home within the confines of the Cornell Electron Storage Ring (CESR), a bastion of linear collider studies at that time. After several years of tests and improvements, the work led to the successful demonstration of an incoherent diffraction radiation beam size monitor in an electron storage ring [3].
Driven by this success, in 2016 the team set their sights on the Advanced Test Facility 2 at KEK, where vertical beam sizes could be compressed to sub-micron levels. Precision became paramount as ultra-thin silicon slits, some with apertures as fine as 40 microns, were crafted and deployed. By 2020, the fruits of their labor materialised as vertical beam size measurements as precise as 5 microns were attained using incoherent UV diffraction radiation [4].
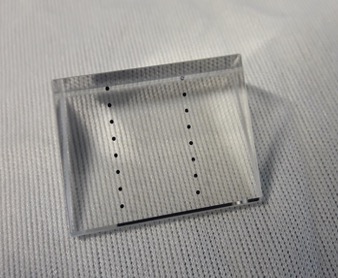
In 2016, confronted with challenges posed by excessive synchrotron radiation background, a novel concept emerged—a shift from thin slits to thicker dielectrics. Thus, the use of incoherent Cherenkov diffraction radiation (ChDR) emerged as an innovation. Its unique attributes promised a remedy to limitations observed while using DR: a greater photon flux (proportional to the length of the radiator) and reduced susceptibility to background interference as radiation is emitted at a well defined large angle. Modifications of the CESR’s test stand were performed and, in 2018, the first incoherent Cherenkov diffraction radiation photons were produced as reported here [5]. This milestone opened new perspectives, systematic studies were performed and horizontal beam profiles measured non-invasively in CESR [6]. However, the typical horizontal beam size in CESR was too large (>200microns) to be conclusive on the real potential of this method.Another shift in focus became necessary prompting a return to ATF2 to explore the resolution limits of ChDR for beam size measurements. In 2020, the unforeseen hiatus imposed by the COVID-19 pandemic perturbs our initial plans. Today, the journey continues and is planned to resume at KEK, poised to adapt to the evolving landscape of Future Circular Collider (FCC) beam parameters.
Moreover, the successful demonstration of Cherenkov Diffraction Radiation in the incoherent domain catalysed a wave of curiosity, prompting exploration into its applicability across diverse beam diagnostic domains. From non-invasive short bunch length monitoring at the CLEAR facility in CERN and the CLARA facility at Daresbury Laboratory, UK [8] to selective electron beam sensing at the proton-driven plasma acceleration facility (AWAKE) at CERN [9], the ramifications of this innovation reverberate across the scientific landscape.
Indeed, the path of research and development is a long journey, a mix of excitement and frustration, breakthroughs and setbacks. Yet, it is sustained by the unwavering dedication and tireless efforts of a cohort of Ph.D. students and post-doctoral fellows, who never stopped pushing forward ... To Lorraine, Thomas, Michele, Robert, Daniel, Alessandro, Kirill, Eugenio, Kacper, Can, Collette, Beth and Andreas.
[1] S.T. Boogert et al, ‘Micron-scale laser-wire scanner for the KEK Accelerator Test Facility extraction line’, Phys. Rev. ST Accel. Beams 13, 122801 (2010)
[2] P. Karataev et al., ‘Beam-Size Measurement with Optical Diffraction Radiation at KEK Accelerator Test Facility’, Phys. Rev. Letters 93, 244802 (2005)
[3] L. Bobb et al. ‘Feasibility of diffraction radiation for noninvasive beam diagnostics as characterized in a storage ring’, Phys. Rev. Accel. & Beams 21, 032801 (2018)
[4] M. Bergamaschi et al., ‘Noninvasive Micrometer-Scale Particle-Beam Size Measurement Using Optical Diffraction Radiation in the Ultraviolet Wavelength Range’, Phys. Rev. Applied 13, 014041 (2020)
[5] R.Kieffer et al. ‘Direct Observation of Incoherent Cherenkov Diffraction Radiation in the Visible Range’, Phys. Rev. Letters, 121, 054802 (2018)
[6] R. Kieffer et al., ‘Generation of incoherent Cherenkov diffraction radiation in synchrotrons’, Phys. Rev. Accel. & Beams 23, 042803 (2020)
[7] A. Curcio et al, ‘Noninvasive bunch length measurements exploiting Cherenkov diffraction radiation’, Phys. Rev. Accel. & Beams 23, 022802 (2020)
[8] E. Senes et al., ‘Selective electron beam sensing through coherent Cherenkov diffraction radiation’, submitted to Phys. Rev. Research (2024)